Between 1992 and 2013, we developed various generic methods to prepare block and graft copolymer nano- and micro-structures. Using these methods, we have pioneered the preparation of many nanostructures that include block copolymer nanofibers and nanotubes. Our research focus is now shifting towards applications of polymer nanostructured materials. Below are our current research directions:
NANOGLIDE Coatings
We have invented a method to nanoengineer coatings that bear nanoreservoirs of a grafted liquid polymer and omniphobicity-enabling surface chains (NANOGLIDE). These coatings repel water, oil, and other laboratory liquids with surface tensions above 20 mN/m. For example, octane droplets at the size of 5 uL glide cleanly down these coatings at a substrate tilting angle < 3o. On them ink and paint traces readily shrink. Moreover, the coatings can be made optically clear at thicknesses up to 100 um and maintain their omniphobicity after extensive surface damage and wear. Further, they can be produced inexpensively, are fluorine-free, and adhere well to a wide range of substrates. Thus, these coatings have many potential applications. We are collaborating with industry to develop anti-graffiti and anti-fingerprint coatings. The anti-fingerprint coatings will be particularly valuable for handheld electronic devices. We are also pushing the boundaries of these coatings and our design principles.
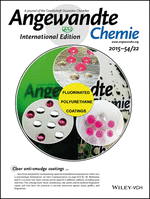
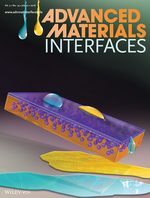
Selected Publications:
- Angewandte Chemie International Edition 2015, 54, 6516-6520
- Angewandte Chemie International Edition 2015, 54, 12722-12727
- Advanced Materials Interfaces 2016, DOI:10.1002/admi.201600001
- ACS Applied Materials & Interfaces 2017, (10), pp 9029–9037
- ACS Applied Materials & Interfaces 2017, 9, 25623 - 25630
Functional Textiles
We have developed various monolayer technologies to coat fibers of textiles. The resultant functional fabrics are then used for applications. For example, functional cotton fabrics have been used to separate oil quickly and cleanly from oil-in-water emulsions. This task was achieved despite the fabric pore sizes of 200 um and oil droplet sizes of < 5 um. We are now scaling up these separations so that this technology can be used industrially. Additionally, we are developing functional fabrics for other applications.
Selected Publications:
- Angewandte Chemie International Edition 2015, DOI: 10.1002/anie.201507451
- Angewandte Chemie International Edition 2016, DOI: 10.1002/anie.201607581
- Angewandte Chemie International Edition 2017, DOI: 10.1002/anie.201706158
Other Nanomaterials
We also prepare other nanomaterials for applications. For example, we recently reported the first preparation of stable encapsulated air nanobubble in water. We are now exploring the applications of these nanobubbles in drug delivery and ultrasound diagnostic applications. We are also developing the next generation of additives to reduce the friction of automobile engine oils. Further, we are developing transparent polymer/metal electrodes that will one day replace indium tin oxide in the electronics industry.
Selected Publication:
- Angewandte Chemie International Edition 2015, 54, 14291–14294
Methodology Development
We continue to develop general methodologies for making nanostructures from block and graft copolymers. Specific activities in this area include:
Micellization
We study BC micellization. For example, we studied the micellization of PA-b-PB-b-PC triblock copolymers and established conditions for obtaining micelles with exotic shapes such as twisted cylinders, tubes, segmented cylinders, hamburgers, and vesicles. The vesicles and tubes were amazing because their PA and PC coronal chains were preferentially localized on the inner and outer wall surfaces, respectively. More interestingly, we discovered generic conditions for BCs to self-assemble into biomimetic double or triple helices.
Selected Publications:
- Journal of the American Chemical Society 2008, 130 (11), 3236-3237
- Macromolecules, 2008, 41 (24), 9727-9735 2016
- Angewandte Chemie International Edition, 2009, 48 (33), 6144-6147
Chemical Processing
We invent general post-self-assembly strategies for preparing BC architectures. To produce “permanent” nanostructures, we selectively crosslink certain domain(s) of self-assembled BC micelles or block-segregated solids. The “permanent” structures turn into “permanent sculpted” nanostructures after we subsequently etch other domain(s). When we first began to chemically process or derivatize self-assembled BC micelles and solids, there had been only 6 papers on this topic. Now, chemical processing is widely used by academic scientists and forms the foundation of various BC nanolithographic processes.
Selected Publications:
- Macromolecules, 1996, 29 (7), 2487-2493 2015
- Advanced Materials, 1997, 9 (5), 437-439
- Advanced Materials, 1998, 10 (1), 69-71
- Angewandte Chemie International Edition 2000, 39 (2), 340-344
- Angewandte Chemie International Edition, 2001, 40 (19), 3593-3596
Coupled Nanostructures and Tier-2 Assembly
The “permanent” and/or “permanent sculpted” BC nanostructures are covalently-linked by us to create unparalleled coupled nanostructures. For example, we end-coupled hydrophobic and hydrophilic nanotubes to yield nanotube multiblocks. They were then further assembled by us at the tier-2 level in a selective solvent to produce “super-micelles”. In a super-micelle, molecular ordering is realized on both the nanometer and the micrometer scale.
Selected Publications:
- Journal of the American Chemical Society, 2003, 125 (46), 14039-14045
- Journal of the American Chemical Society, 2004, 126 (32), 10059-10066
- Journal of the American Chemical Society, 2006, 128 (49), 15921-15927
Directed Assembly
In another post-self-assembly strategy, we combine BC self-assembly with emulsification to perform directed assembly. Emulsification is used to create confined and oil-water interfaces as sites for BCs to self-assemble. Directed assembly yields “cell-like” and “virus-like” particles and opens the doorways to sophisticated biomimetic structures.
Selected Publications:
- Nano Letters, 2001, 1 (12), 683-687
- Journal of the American Chemical Society, 2005, 127 (44), 15358-15359
- Journal of the American Chemical Society, 2008, 130 (11), 3236-3237
BC/Inorganic Hybrid Architectures
Aside from BC architectures, we create BC/inorganic hybrid architectures. We have produced inorganic nanoparticles inside BC templates, generated inorganic particles using a BC as the stabilizer (surfactant), or coated pre-formed inorganic particles by a BC. Inorganic particles are produced mainly to render function and applications to the final hybrid structures.
Selected Publications:
- Chemistry of Materials, 2005, 17 (24), 6053-6059
- ACS Nano, 2008, 3 (1), 165-172
Architectural Polymers
Our latest methodological breakthrough is in the chemical processing of unimolecular micelles and associated block copolymer dimers to prepare architectural polymers. Unimolecular micelles are formed in block-selective solvents below the BC’s critical micellar concentrations (cmc). Since the cmc is typically very low for a BC, unimolecular micelles had been ignored in the past. We invented a method to promote unimolecular micelle formation and subsequently chemically locked their structure to yield architectural polymers. Tadpoles, imprinted tadpoles, and macrocycles have thus been produced. While a tadpole consists of a polymer chain tethered to a crosslinked nanobead, the bead of an imprinted tadpole is molecularly imprinted with high chiral selectivity. Large macrocycles have been traditionally difficult to prepare at high concentrations and in high purity. Our method overcame all of these challenges. The above “assemble-and-then-react” method has also been used by us to prepare mikto-arm copolymers. In this case, different block copolymers are first associated into dimers, and the dimer structure was then locked by a chemical reaction.
Selected Publications:
- Macromolecules 2008, 41 (15), 5697-5702
- Macromolecules 2014, 47 (15), 5096-5103